Click on the Course name to view the speaker(s) and abstract.
1-Hour Q&A (Watch Pre-Recorded Video Prior)
Speakers: Olivier Couture, Vincent Hingot
Super-resolution imaging has the capacity to distinguish and map structures that are smaller than the classical limit, typically a fraction of the wavelength. For ultrasound imaging, this means exploring features, such as blood vessels, in the micrometric range deep inside tissue. At the end of this course, students should be able to understand and reproduce super-resolution ultrasound imaging experiments, from data acquisition to image reconstruction, and apply such knowledge in their specific fields. We first explore the fundamental aspects of imaging resolution in ultrasound. Various approaches to bypass the diffraction-limit with microbubbles and other agents are presented. More particularly, we discuss ultrasound localization microscopy, which has recently improved the resolution for vascular imaging by more than 10-fold. We present its various steps, including separation, localization and tracking, and compare different approaches. Specific elements such as temporal resolution, motion correction or volumetric imaging are considered. We then detail the applications of super-resolution ultrasound for brain, tumor, kidney, liver lymph nodes and peripheral vessels imaging, along with future perspectives in the clinical and preclinical context. The last part of the course will include hands-on image processing of in-silico and in-vivo open data with provided ultrasound localization microscopy algorithms.
1-Hour Q&A (Watch Pre-Recorded Video Prior)
Speakers: Thomas Szabo, Peter Kaczkowski
This introductory course is intended to give students and those newly entering the field of ultrasound imaging an overview of medical ultrasound. We provide a framework for introducing the concepts of how an ultrasound system images the body and how it can be applied to different applications. First, we present how tissues interact with sound waves through a series of simulators. Second, we follow signals through an imaging system as they turn from electrical pulses in a beamformer and transducer into acoustic waves and back into electrical signals and finally into an image. Third , we demonstrate different types of imaging modes and their role in the visualization of three-dimensional objects. Fourth, we explain how an ultrasound research system can be reconfigured to serve different functions and applications. Finally we provide a brief overview of the different branches and specialized applications of ultrasound. Throughout the course, we demonstrate the principles by using special purpose interactive computer simulators as well as live experiments and imaging with an ultrasound research system.
3-Hour Live
Speakers: Yonina Eldar, Ruud JG van Sloun
Over the past years, deep learning has established itself as a powerful tool across a broad spectrum of domains. While deep neural networks initially found applications in the computer vision community, they have quickly spread over medical imaging applications, ranging from image analysis and interpretation to – more recently – image formation and reconstruction. Deep learning is currently also rapidly gaining attention in the ultrasound community. This course will first cover the basic principles of deep learning, ranging from understanding the relevance of sequential nonlinear transformations for representation learning to log-likelihood based optimization of neural network parameters. Optimization aspects such as the impact of local minima and saddle points in the solution space will also be touched upon. We will then discuss the design of effective neural network architectures, including dedicated solutions that e.g. leverage signal structure through unfolding methods. The second part will focus on the wealth of opportunities that deep learning brings for ultrasound imaging. Beyond image-level classification and segmentation, we will discuss neural networks for front-end receive processing, including beamforming, clutter suppression, and advanced applications such as super-resolution imaging.
4-Hour Live
Speaker: Mickael Tanter
The advent of ultrafast ultrasonic scanners is paving today the way to tremendous applications in medical Ultrasound. This course will present the basic principles of Ultrafast Imaging (plane or diverging wave imaging, parallel receive beamforming, coherent plane wave compounding, …) and their implications in terms of resolution, contrast and frame rates. It will also explain the analogy such concept with optical holography. For our purposes, theoretical aspects and experimental validations will be highlighted. The course will also emphasize technological issues and system architecture constraints. Far beyond breaking technological barriers, this concept of ultrafast imaging is currently changing the paradigm of ultrasound imaging. The course will illustrate how this concept leads to breakthrough innovations in the field by revisiting Bmode, Doppler, tissue strain and nonlinear imaging. Many examples (Shear Wave Imaging, Ultrafast Doppler, fUltrasound, Ultrafast Contrast Imaging, ultrafast Ultrasound Localization Microscopy,…) will illustrate the potential of this paradigm shift in ultrasound imaging.
1-Hour Q&A (Watch Pre-Recorded Video Prior)
Speakers: Marcin Lewandowski, Billy Yiu, Piotr Jarosik, Mateusz Walczak, Piotr Karwat, Ziemowit Klimonda, Julia Lewandowska
Nowadays, GPUs (Graphics Processing Units) serve as work-horses for processing massive amount of data and to accelerate general-purpose scientific and engineering computing.
The main goal of the training is to get familiar with the GPU/parallel programming and apply it to ultrasound signal processing. The short-course is going to be practically oriented with a 50/50 split between the lectures and exercises. We are planning to leverage a common knowledge of the basic ultrasound processing methods and show how to translate them into working parallel algorithms.
The workshop will target both low-level Nvidia CUDA GPU programming and high-level Python tools. This blend of development tools enable fast prototyping of new processing methods and later migration to a high-performance native GPU implementation. During the exercises, the Participants will implement and test their algorithms on ready to use RF datasets, as well as have an opportunity to run them on an ultrasound research system equipped with GPU.
1-Hour Q&A (Watch Pre-Recorded Video Prior)
Speaker: Damien Garcia
Motion estimation by ultrasound imaging plays an important role in clinical diagnosis. It mainly involves the estimation of blood velocity and tissue displacements. This course will provide an overview of basic techniques for motion estimation including speckle tracking, color Doppler and vector Doppler. It will be supported by some Matlab demonstrations and a standalone executable program. It will also be illustrated with vascular and cardiac examples. The following topics should be covered:
• Back to basics: RF, I/Q & speckles
• Motions in the cardiovascular system
• Doppler & vector Doppler
• Speckle tracking by block-matching
• Other techniques for motion estimation
• Fast ‘n easy examples using Matlab
• Cardiovascular applications & clinical perspectives
1-Hour Q&A (Watch Pre-Recorded Video Prior)
Speaker: Charles Courtney
Acoustic tweezers are devices that use configurable ultrasonic acoustic fields to trap and then manoeuvre small objects. In order to develop devices and strategies for dexterous manipulation in these devices we must understand: the underlying physics that produces the acoustic radiation force, the acoustic fields that cause trapping and how these fields can be generated in practice.
In this course the physical principles that lead to the Gor’kov equation (which determines the forces on objects much smaller than the acoustic field’s wavelength) will be described and the equation derived. Alternative scattering regimes, for larger objects will also be considered using ray acoustics and numerical modelling. The various methods and devices that have been designed to dexterously manipulate microparticles will be reviewed. Examples of recent applications will be discussed in context of the described theory and methods.
4-Hour Live
Speaker: Alexei Maznev
Optical generation and detection of acoustic waves make it possible to conduct ultrasonic measurements without transducers in a non-contact and non-invasive manner. This approach referred to as laser ultrasonics is particularly well-suited for surface acoustic waves (SAWs), as the surface is typically amply accessible to light, and spatial shaping of the optical intensity at the surface allows for a great degree of flexibility and control. In this short course, we will cover the following topics:
-Physical principles and mechanisms of the optical generation of SAWs.
-Established and emergent experimental techniques for laser generation and detection of SAWs including recent developments such as using extreme ultraviolet light to generate SAWs up to 50 GHz in frequency.
-The use of laser-generated SAWs for studying fundamental wave propagation phenomena including surface phononic crystals and metamaterials as well as nonlinear SAWs and solitons.
-Materials science applications such as characterization of elastic properties of thin films, assessing radiation damage of sub-surface layers, measuring SAW attenuation, and testing material strength under ultrahigh strain rates.
-Potential industrial applications for metrology and process control.
1-Hour Q&A (Watch Pre-Recorded Video Prior)
Speaker: Ji Wang
With the rapid miniaturization of acoustic wave resonators for frequency control and sensor applications, the analysis and design of acoustic wave resonators are moving toward computer-based process with strong demands on formulation and modeling with the consideration of materials and dynamic features. In responding to heavy dependence on design tools, it is important to start with the fundamental theories of acoustic wave devices to support numerical analysis. We present the basic equations of wave propagation in piezoelectric solids with emphasis on device structures, crystal materials, plates, films, and layered structures corresponding to their vibration modes and frequencies. Simplifications are made to typical resonators for their distinctive frequencies in relation to the functioning modes. These results are important in guiding the inception and development of newer types of resonators. Further improvements of analysis can be done along this line by utilizing numerical methods for accurate solutions of vibration modes and frequencies of actual resonator structures for optimization to achieve better performance and accurate extraction of electrical parameters for circuit design. The formulation and methods can be applied to common types of resonators such as quartz crystal resonators (BAW), surface acoustic wave resonators (SAW), and film bulk acoustic wave resonators (FBAR). Eventually, a formal procedure of the complete formulation and approximate analysis of acoustic wave resonators will be presented and discussed. This course is considered as the introduction of the formulation and analysis of performance properties of acoustic wave resonators, and further engineering solutions can be acquired with the help of practical tools such as finite element analysis.
4-Hour Live
Speaker: Yook-Kong Yong
Today’s precision piezoelectric acoustic wave devices require many common features such as high Q, low power, small size, and stringent requirements on frequency stability, temperature stability, and force sensitivity. Since the devices are employed as elements of frequency standards and detection, their frequency performances have to be maintained by precision designs, manufacturing, and operations. Consequently, the analysis and design of these piezoelectric devices would need 2-D or 3-D models that are accurate in terms of the resonator geometry, mountings and material properties. Furthermore, we would also need models for nonlinear analysis that include effects such as (1) temperature sensitivity, (2) applied forces from environmental vibrations, (3) harmonic generation, and (4) intermodulation. These models are useful for design and analysis of acoustic resonators because we have been successful in extracting their electrical circuit parameters and identifying the major factors impacting their precision frequency performances. The course will first focus on the primary aspects of accurate linear finite element modeling, such as the frequency spectra and quality factor Q as functions of resonator geometry and mountings. Comparisons of model results with the relevant experimental results are presented. Next the nonlinear finite element modeling of these devices are discussed. Both linear and nonlinear material properties and deformations are taken into account. The linear and nonlinear material constants for common piezoelectric materials are discussed and presented. The nonlinear behavior of quartz resonators such as their frequency-temperature behavior, force-frequency effects, and nonlinear resonance including the Duffing effect will be presented. If time permits, nonlinear frequency response modelling for force frequency effects, harmonic generation and intermodulation of BAW and SAW resonators will be presented and compared to experimental results.
1-Hour Q&A (Watch Pre-Recorded Video Prior)
Speaker: Tao Han
With the rapid development of surface acoustic wave (SAW) technology, SAW devices with complex structures incessantly emerge in filtering and sensing application. The temperature frequency characteristics, the packaging on the stability of SAW filters, and the sensing mechanism analysis of SAW sensors inevitably involve the mechanical-electro coupling characteristics of SAW resonators.
Based on the quick overview of perturbation theory on SAW devices in biasing fields, this short course mainly focuses on the SAW electro/magneto/solid mechanical/thermal multi-physics coupling model. The electric and solid mechanical fields are strongly coupled by piezoelectric constitutive equations. The other multi-physical fields, such as the thermal, the stress, the magnetic fields and so forth can be considered as increment biasing fields using the nonlinear perturbation theory. Therefore, combinations of direct coupling and sequential coupling are established in the SAW multi-physics coupling model. Effective material constants are functions of the heterogeneous multi-physics fields at different mesh nodes. Nonlinear constitutive equations and complicated boundary conditions are modified using weak form expressions with the combination of the contributions of biasing stress and strain tensors, as well as the influence of the third-order material constants of the substrate, electrodes, and layers. Taking SAW pressure sensors at high temperatures, magnetic field sensors and TC-SAW, IHP-SAW resonators as examples, the modelling of multi-physical fields coupled SAW resonators are introduced. The simulation and experimental results are presented and in good agreement with each other.
4-Hour Live
Speakers: Songbin Gong, Shuji Tanaka
MEMS and acoustic wave (SAW and BAW) devices are based on similar technology and physical background, but they have been developing in different communities for a long time. In this short course, the lecturers, who have been deeply involved in developing both MEMS and acoustic wave device technologies, present and discuss the following MEMS-related design and implement techniques for the ultrasonics community:
1. Design techniques for RF devices enabled by wafer-bonding and layer transfer
2. MEMS fabrication techniques for acoustic wave devices
In the first half of this short course, topic 1 will be discussed by Prof. Gong, introducing several device-level design techniques to best harness the performance out of transferred single- crystal piezoelectric layers. Both resonant (e.g. resonators and filters) and non-resonant devices (delay lines and correlators) will be showcased, and their design spaces will be explained. It will be shown that the device platforms based on transferred single crystal piezoelectric layers hold strong potential for a wide range of RF applications from 5G to IoT.
In the second half of this short course, topic 2 will be lectured by Prof. Tanaka. Using an acoustic wave device as an example, the general fabrication process is explained. Simultaneously, the fundamental and know-how of fabrication techniques such as deposition, etching, wafer bonding and wafer-level packaging will be explained. The goal of the first half part is to understand practical MEMS fabrication technology and to widen attendees’ options of fabrication and packaging methods for various acoustic wave devices.
4-Hour Live
Speakers: Scott Smith, David Mills
Ultrasound has become the most commonly performed medical imaging procedure in the world because it provides real-time imaging with high clinical value while being portable, non-ionizing and inexpensive. This course will provide an introductory survey of ultrasound imaging focused on the design, fabrication, and testing of medical ultrasound transducers. Starting from an overview of the basic types of phased-array transducers (linear, convex, sector), we will show how the probe’s design is derived from its target application. We will describe how engineering tools, like equivalent-circuit, finite-element, and acoustic field models, can be used to predict transducer performance accurately, and then to optimize the design. A discussion of the structure of an ultrasound probe will lead to a survey of the different types of materials used in probes and their critical properties. Typical fabrication processes will be reviewed and common problems in probe manufacturing will be summarized. Methods for evaluating completed transducers will be described. The course will include recent developments in probe technology, including single crystal piezoelectrics, cMUT transducers, catheters, 2D arrays, and electronics in probes, and will address some of the performance advantages and fabrication difficulties associated with them.
4-Hour Live
Speakers: Susan Trolier-McKinstry, Sandy Cochran
Piezoelectric ultrasound transducers are ubiquitous in contemporary ultrasound systems, with applications including biomedical therapy and imaging, nondestructive evaluation, and underwater sonar. This course provides a foundation of understanding of the fundamentals of piezoelectric materials and their use in ultrasound transducers, and an informed appreciation of more advanced subjects in the state of the art.
Piezoelectric single crystals, ceramics, and thin films are introduced, along with mathematical descriptions of them and their behavior and an explanation of the underlying physics. The nature of different materials is described, with particular reference to the phase diagrams, domain structures, and the resulting impact on properties. Comparisons will be made between single crystals and ceramics, with an emphasis on the impact on parameters essential for ultrasound generators and receivers.
The operating principles of transducers based on piezoelectric materials are described with reference to wave propagation within and external to the transducer. Electrical impedance spectroscopy is introduced for both material characterization and transducer performance prediction, with emerging characterization techniques and virtual prototyping highlighted.
Ultrasound transmission and reception techniques are considered, and these will be linked with modelling, through descriptions of one-dimensional models and a summary of the capabilities of finite element analysis. The effects of different piezoelectric materials are demonstrated with simplified transducer configurations and the selection of materials for specific applications are considered in detail.
Throughout the course, practical examples and modeling are used to illustrate the concepts under discussion, established and new piezoelectric materials, characterization and analysis, and how piezoelectric components affect transducer behavior.
1-Hour Q&A (Watch Pre-Recorded Video Prior)
Speakers: Erdal Oruklu, Jafar Saniie
In this short course, we present machine learning and signal processing algorithms for ultrasonic imaging applications. This course covers several case studies such as detecting defects in critical components used in nuclear power plants, pulse-echo chirplet estimation, flaw detection in large-grained materials using order statistics and deep artificial neural networks, ultrasonic data compression using machine learning, software-defined ultrasonic system design for communication through solid structures, and hardware/software codesign using system-on-chip for ultrasonic signal processing applications.
Short Course Schedule
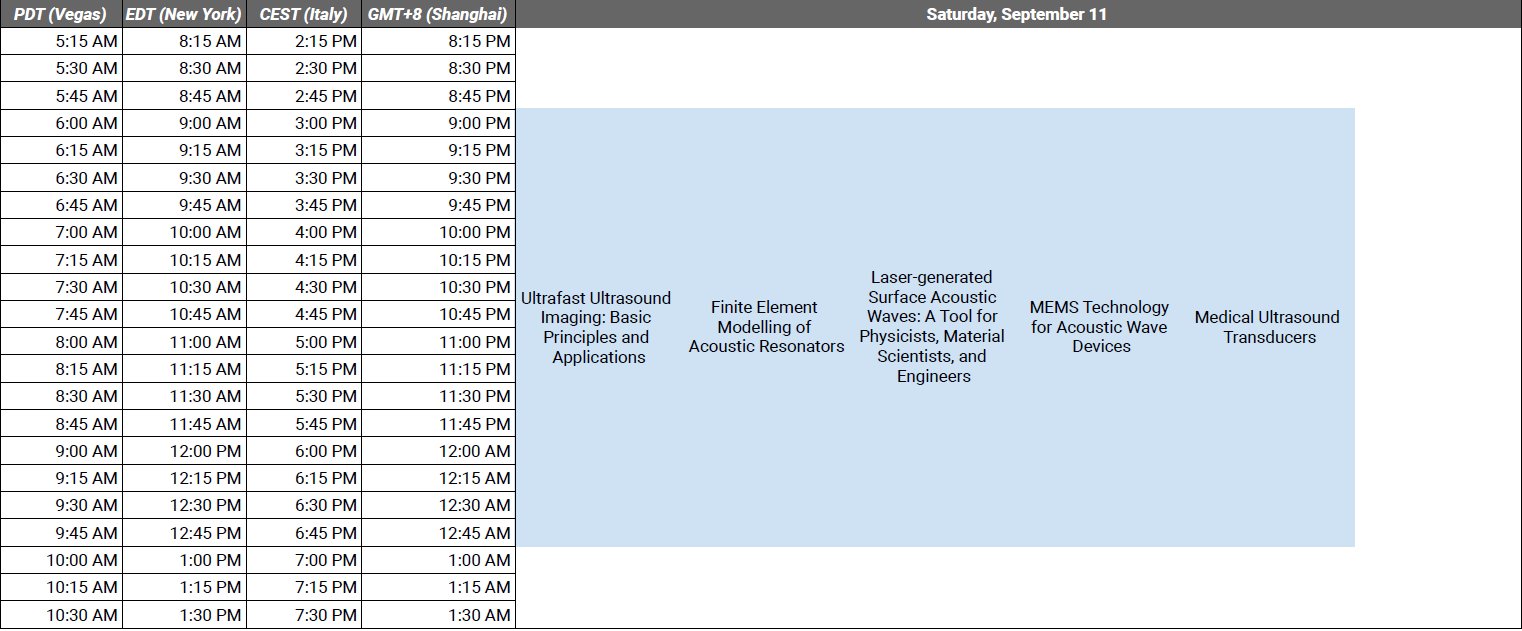
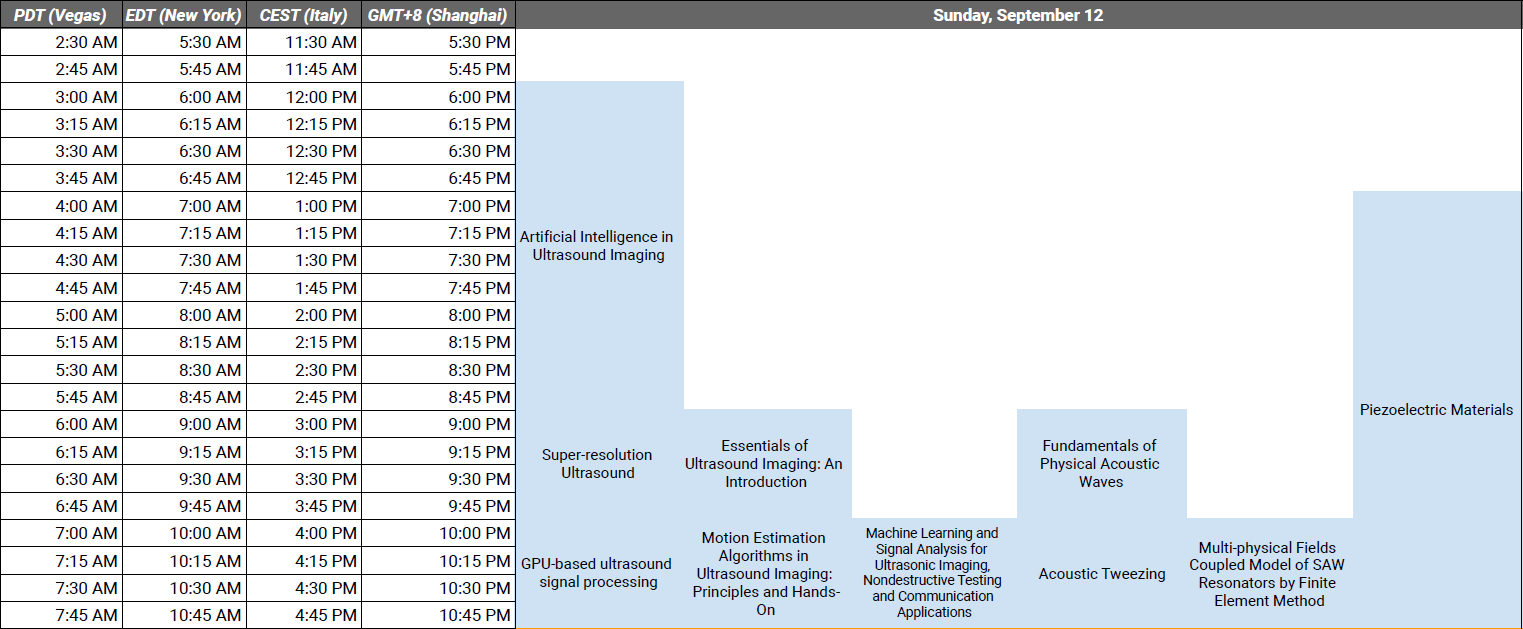